Parameter Optimisation
What parameters impact in research and development of biological processes?
Bioreactors, as specialized vessels designed to provide optimal conditions for biological processes, allow the fine-tuning of key parameters. Screening of the optimal parameters is fundamental in bioprocessing as it directly impacts the performance and productivity of biological systems. Bioreactors play a crucial role in this optimization by providing a controlled environment where key parameters such as temperature, pH, oxygenation, nutrient supply, and agitation can be precisely regulated. By optimizing these parameters, researchers can enhance cell growth, metabolism, and product formation, leading to increased yields, improved quality, and efficient scaling up of bioprocesses. Culture conditions also will directly impact culture morphology and physiology, but also will after which secondary metabolites are produced. Bioreactors are invaluable tools for parameter optimization, enabling researchers to achieve optimal conditions and reliable results in various industries.
Temperature
Temperature directly influences organisms’ growth and metabolic activity, and therefore it will impact the product formation of cells, whether it is metabolites or biomass. The optimization of temperature is thus crucial.
Temperature plays a significant role in cell growth kinetics; optimal values depend on the organism. Deviations from the value result in suboptimal growth rates, extended lag phases, and cell death. Precise adjustment and maintenance of the temperature with a favorable range promotes rapid and efficient cell growth, leading to higher biomass production.
The temperature has an impact on chemical and biochemical reactions and, therefore, will impact enzymatic activity and metabolic pathways. Enzymes exhibit temperature optima at which activity is highest. The fine-tuning of temperature can help to ensure that conditions remain within the ideal range, enhancing enzymatic activity and facilitating the desired biochemical reactions within the bioreactor.
Proteins and other biomolecules’ structural stability and functionality depend highly on temperature. Changes in the value can lead to protein denaturation and loss of activity. Fine control of temperature can ensure structural integrity and functionality of targe biomolecules, maximizing their effectiveness in the bioprocess.
In summary, temperature optimization in bioprocesses is vital for promoting optimal cell growth, enhancing enzymatic activity, and maintaining protein stability. By carefully controlling and adjusting the temperature within the bioreactor, researchers can create an environment that maximizes the desired biochemical reactions, leading to improved productivity, yield, and overall process performance.
pH
Medium acidity or alkalinity greatly impacts bioprocess optimization, and precise control of this parameter is crucial for cell growth, metabolism, and product formation.
Enzymatic activity, and therefore metabolic pathways, are strongly dependent on pH. Enzymes exhibit specific pH optima at which their activity is maximized. Deviation from this value results in reduced enzymatic efficiency and, consequently, reduced metabolic activities. By precisely adjusting and maintaining the pH within the optimal range, enzymatic activity can be enhanced, yielding the desired products.
A biomolecule’s structure and stability are heavily influenced by pH due to charge distribution, which determines the conformation of proteins and nucleic acids. Optimization of pH ensures that the aforementioned structures are stable.
Similarly to enzymes, cells have narrow pH ranges at which their growth is optimal. This range will depend on the organism, and variation in this value can be detrimental to the growth due to changes in membrane permeability, nutrient update, and waste product excretion.
Organic compounds can have different solubility values and speciation under different pH values, affecting their bioavailability which can impact cell uptake.
In summary, pH optimization in bioprocesses is critical for maximizing enzymatic activity, preserving biomolecular stability, promoting cell growth, and optimizing substrate availability. By carefully adjusting and maintaining the pH within the appropriate range, researchers can create an environment that facilitates the desired biochemical reactions, improving bioreactors’ productivity, yield, and overall process performance.
Oxygenation
Oxygen concentration is a vital parameter in bioprocess optimization for aerobic (oxygen-dependent) and anaerobic (oxygen-independent) organisms. The volumetric mass transfer coefficient of oxygen (KLa) determines the concentration of oxygen in the medium. This coefficient shows how efficiently oxygen transitions from the gas phase into the liquid phase. In the case of aerobic organisms, oxygen is used for cellular respiration and energy generation; therefore, ensuring an adequate supply is fundamental. On the other hand, oxygen is detrimental for many anaerobic organisms, resulting in slower processes or even cell death. In some processes, the metabolic pathways that would produce target biomolecules are heavily regulated by the presence of oxygen (e.g. facultative fermentations), and a fine control of the oxygen values is crucial.
In some cases, excessive oxygen levels or prolonged exposure to this gas can cause oxidative stress, which results in oxidative damage to biomolecules and cells. Striking the balance between oxygen demand and avoiding oxidative effects can help ensure optimal cell performance.
Furthermore, oxygenation affects mass transfer rates within the bioreactor, influencing the availability of other dissolved gases, nutrients, and waste removal. Optimization of oxygenation parameters, such as agitation speed, aeration rate, or sparging methods, enhances mass transfer efficiency, reducing concentration gradients and ensuring optimal cell growth and product formation.
Optimizing oxygenation in bioprocesses is crucial for facilitating aerobic metabolism, supporting cell growth and viability, and promoting the synthesis of desired products.
Agitation and mixing
Agitation and mixing are critical parameters in bioprocess optimization, ensuring efficient mass, energy transfer, and homogeneous distribution of nutrients and gases. Agitation and mixing are controlled by the design and configuration of the agitation system, such as impeller design, speed, and presence/absence of baffles. These factors influence the rate and pattern of mixing within the bioreactor. Careful selection and optimization of agitation patterns contribute to achieving desired mixing levels, preventing the formation of dead zones or excessive shear forces.
Effective mixing ensures all cells have equal access to nutrients, promoting uniform growth and preventing localized nutrient depletion. Adequate oxygen distribution is fundamental for aerobic processes, preventing anaerobiosis, which results in suboptimal functioning and, eventually, cell death.
Appropriate mixing prevents concentration gradients – nutrient availability, pH, dissolved gases, or waste product accumulation. These gradients can be detrimental to cell growth, metabolism, and product formation. By creating turbulence and promoting fluid movement, contact between cells and the surrounding medium improves, resulting in better nutrient uptake.
Agitation processes heavily impact the temperature inside the reactor. Mixing helps distribute heat from external suppliers, such as heating jackets, and the heat generated by metabolic processes. Localized heat accumulation results in cells stress, impacting growth and metabolic processes.
Nutrient supply
The medium composition and nutrient supply are vital components for optimizing bioprocesses, ensuring that all the essential substances for cell growth, metabolism, and productivity are supplied. The optimization of the medium includes carbon and nitrogen sources and macro and micronutrients. Bioreactors can operate different operation modes, such as batch, fed-batch, or continuous feeding. The selection of the appropriate strategy will impact product formation and growth rate. Optimizing feeding strategies ensures a continuous and balanced nutrient supply throughout the bioprocess, maintaining favorable growth conditions and maximizing productivity.
The possibility of adding measuring probes allows for real-time process monitoring and control, ensuring an optimal nutrient supply. Feedback control systems are utilized to maintain nutrient concentrations within desired ranges, ensuring consistent nutrient supply and promoting optimal cell growth and product formation.
In summary, nutrient supply optimization in bioprocessing encompasses various factors, including selecting and concentrating carbon and nitrogen sources, the provision of macronutrients and micronutrients, media composition, nutrient feeding strategies, process monitoring and control, and waste management. By carefully considering these aspects, researchers can create an environment that supports optimal cell growth, metabolism, and productivity, leading to successful bioprocesses within bioreactors.
Solutions
The BioXplorer, one of the flagship products at H.E.L, is incredibly powerful for bioprocess optimization. Its customization capability allows one to choose the probes for specific requirements, providing unparalleled flexibility and precision. Its integration with H.E.L WinISO enables rigorous control over bioprocess conditions, ensuring optimized and reliable workflows.
Regarding the specialized measurements, we offer auxiliary tools from H.E.L, such as BioVIS or Tandem Off Analyzers, integrating seamlessly with the BioXplorer. All these elements combined underscore H.E.L’s dedication to crafting solutions that exceed industry expectations.
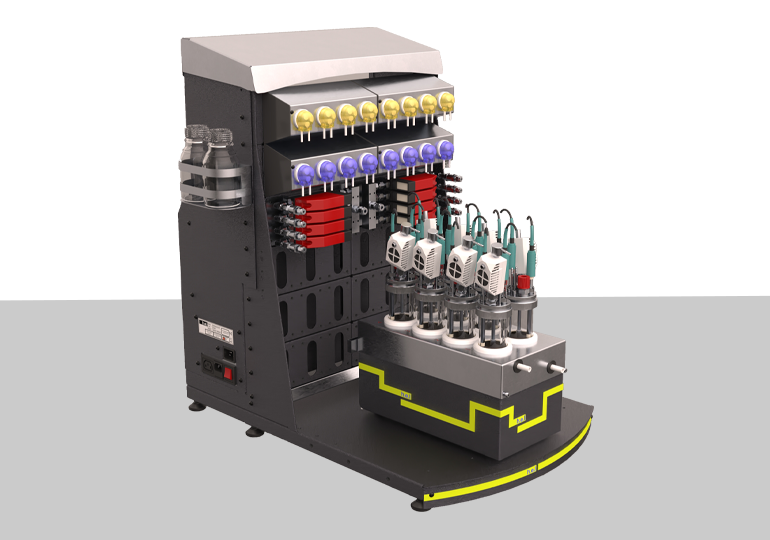
BioXplorer 100 | bench-top, parallel 8 bioreactor platform
The BioXplorer 100 is a multi-bioreactor system designed for the optimization of aerobic f...
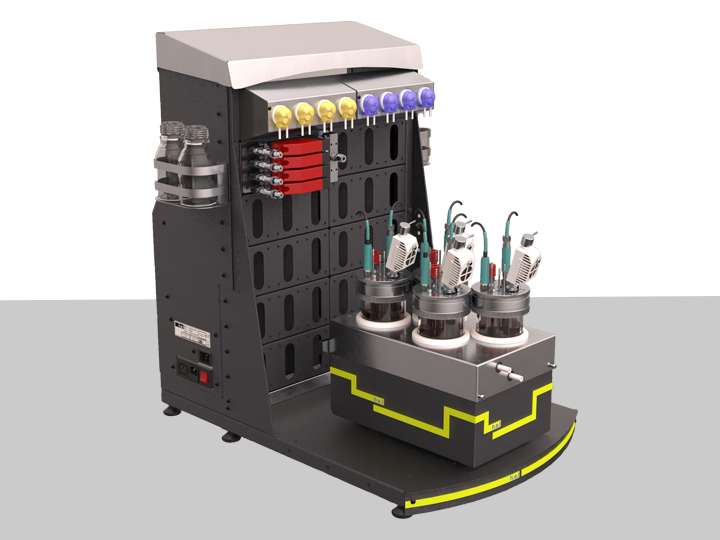
BioXplorer 400 | bench-top, parallel 4 bioreactor platform
The BioXplorer 400 is equipped with 4 bioreactors with working volumes of up to 400 ml. It...
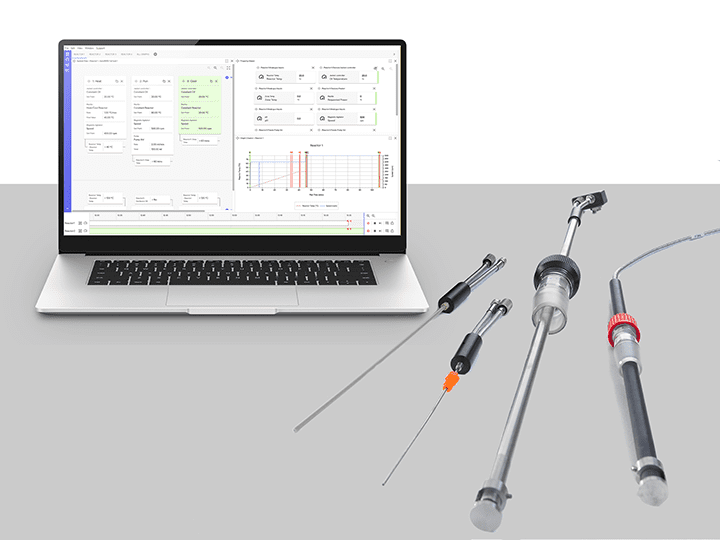
BioVIS | An Inline Cell Density Indicator Sensor
BioVIS is a probe for the inline monitoring of total cell growth and biomass within a bior...
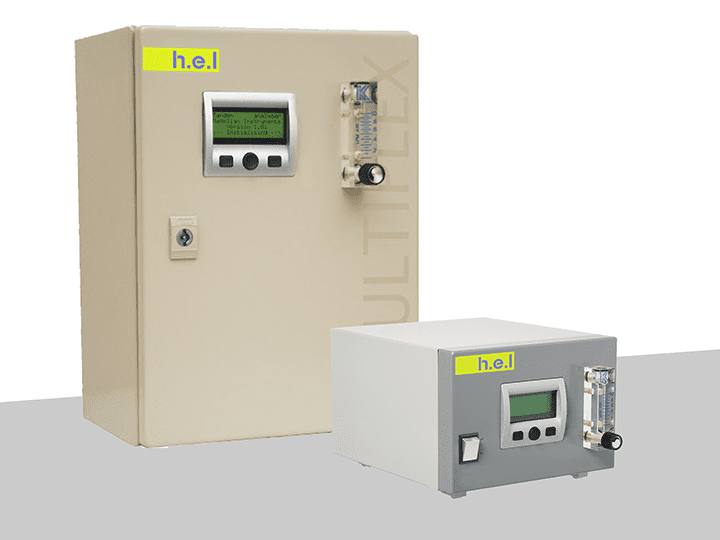
Tandem Off Gas Analyzers | Multi Line and Continuous Systems
The Tandem gas analyzer systems will give you real-time, on-line data that helps you under...
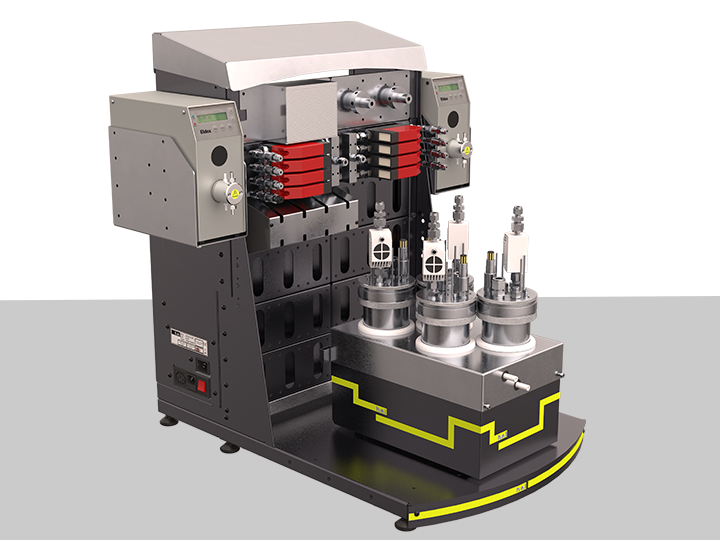
BioXplorer 400P | High-pressure bench-top, parallel 4 bioreactor platform
The BioXplorer 400P is an automated parallel pressure bioreactor system for the discovery ...
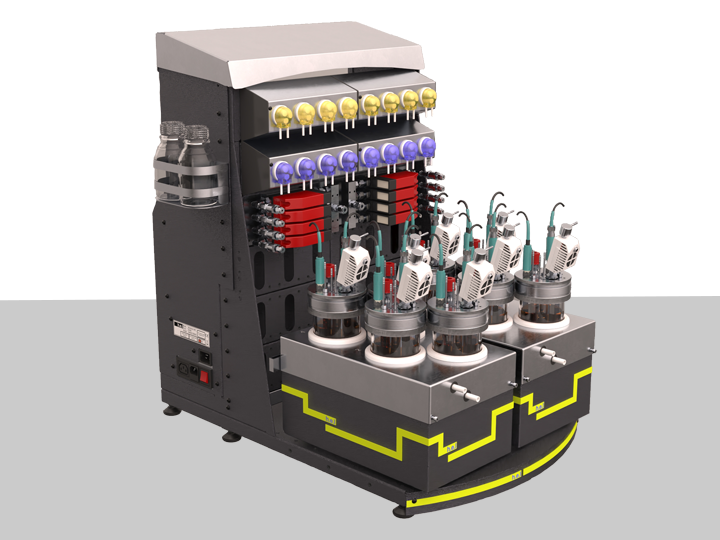
BioXplorer 400XL | Expanded Bench-Top, Parallel 8 Bioreactor Platform
Featuring eight 500 ml bioreactors, the BioXplorer 400XL allows the fast optimization of c...
Screening
What processes can be screened using bioreactors?
Gut microbiome and its responses
The microbiome can be defined as the collection of all microorganisms inhabiting a particular ecosystem. In the case of humans, whose bodies are made from trillions of cells, the ratio between human cells and microbial cells is approximately 1:1. The gastrointestinal system contains the majority of microorganisms that live in association with humans. Health benefits and diseases have been tracked to the gut microbiome, which is involved in processes as diverse as digestive disorders and neurological diseases.
Understanding this microbial ecosystem has been deemed a priority in the last few years, with the creation of networks such as the Human Microbiome Project, or INFOGEST. This type of entity aims to promote research in this field and harmonize the techniques used to do so so results are comparable between studies. Characterization of the microbial community and its responses is not an easy task. From cultivation studies to current metagenomic approaches, knowledge has been accrued in the last few years. Studies on what a healthy and “normal” gut microbiome is have been extensively published, as well as variations in this normality and its potential consequences.
However, bioreactors can be very powerful tools for understanding how the gut microbiome reacts to different factors. INFOGEST, on its recommendations, has produce a list of different simulated fluids (such as salivary, gastric, and intestinal). The strict control and automation of bioreactors enable thorough mixing of its contents. The use of tubing allows for the delivery of the simulated fluids in a staggered fashion, mimicking the digestive process. Additionally, interconnection between reactors in parallel systems can help to pump the simulated bolus, replicating the transposition between the different chambers of the gastrointestinal tract. Approaches like this help to understand how food can be digested over time, or how the microbiome can respond to different diets.
Syngas fermentation
Syngas (synthesis gas) is a mixture of gases, predominantly carbon monoxide (CO) and hydrogen (H2) and other minor gases, such as CO2, methane, and hydrogen, derived from the gasification of carbonaceous fuels and biomass. It can be used as a clean alternative to fossil fuels for the production of added-value products and biofuels.
The transformation process from syngas to the final product is usually carried out by microorganisms. Autotrophic organisms have the ability to generate their own organic matter using inorganic carbon such as CO2, CH4, or CO. Whereas photosynthesis (using light as energy source) is the most known type of autotrophy, chemolithotrophy (using inorganic chemical reactions to produce the energy needed for the process) poses a very interesting alternative with application in syngas fermentations. The oxidation of reduced molecules (such as H2 – present in syngas-, sulfur, nitrogen, or iron) can power the cells that fix the inorganic carbon.
However, the main limitation of gas fermentation, in general, and syngas fermentation, in particular, is the limited solubility of the gaseous phase, limiting its bioavailability. This, in turn, reduces the productivity of the process. One of the best ways to increase solubility is to increase the pressure inside vessels. Bioreactors, especially metal-made ones, can be pressurized, helping to overcome this limitation. An extra concern that some gases can present (e.g., H2) is their potential flammable/explosive characteristics. This issue can be worsened by the need of certain microorganisms for oxygen for their fundamental metabolism. Real-time measurements can help to monitor the concentration of the different reagents involved in the biological reactions. Using tight control measures, such as nitrogen flushing, and feedback loops, can help guarantee that the conditions inside the bioreactor are never close to unsafe values.
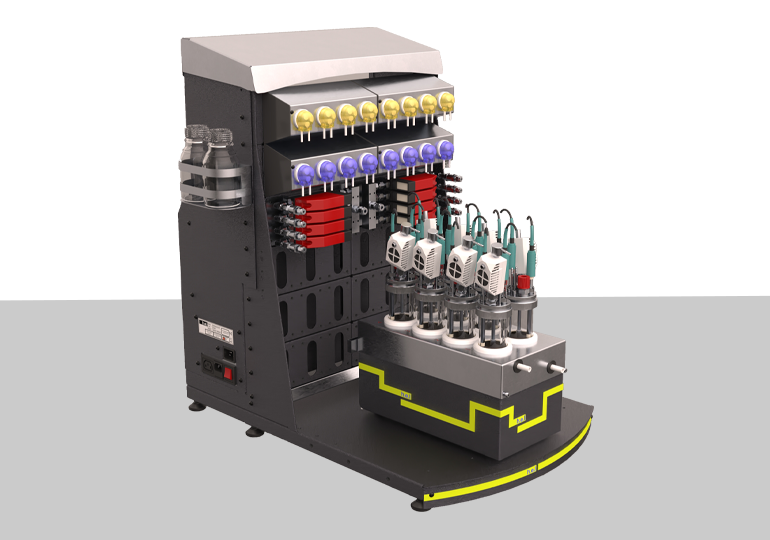
BioXplorer 100 | bench-top, parallel 8 bioreactor platform
The BioXplorer 100 is a multi-bioreactor system designed for the optimization of aerobic f...
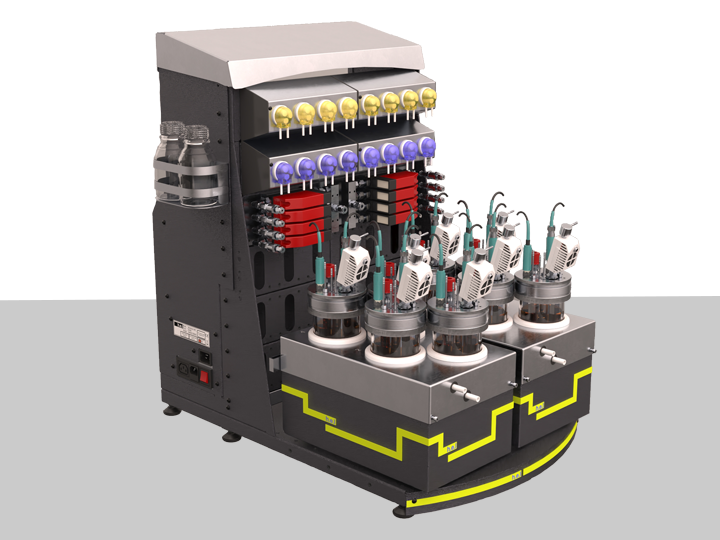
BioXplorer 400XL | Expanded Bench-Top, Parallel 8 Bioreactor Platform
Featuring eight 500 ml bioreactors, the BioXplorer 400XL allows the fast optimization of c...
How can the media feed be optimized?
Different strains have different medium requirements in terms of carbon and nitrogen sources, vitamins, and micro and micronutrients. However, different feeding strategies will also impact the growth and efficiency of cultures.
Batch feeding
This approach adds nutrients to the bioreactor in a single batch. It proves a controlled and defined nutrient environment for the screening process.
Fed-batch feeding
This type of feeding consists of continuous or intermittent feed addition throughout the cultivation process. Fed-batch feeding provides greater control over nutrient availability. Tuning the feed rate, nutrients, and compositions can result in significant growth, productivity, and product quality.
Continuous feeding
This approach consists of the continuous addition of feed of nutrients to the bioreaction at a constant rate throughout the process. This results in a controlled nutrient availability and growth rate, enhancing product performance.
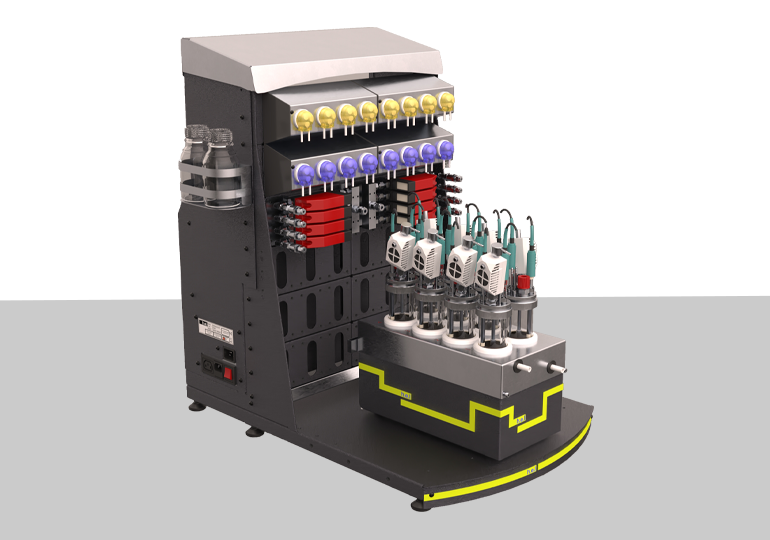
BioXplorer 100 | bench-top, parallel 8 bioreactor platform
The BioXplorer 100 is a multi-bioreactor system designed for the optimization of aerobic f...
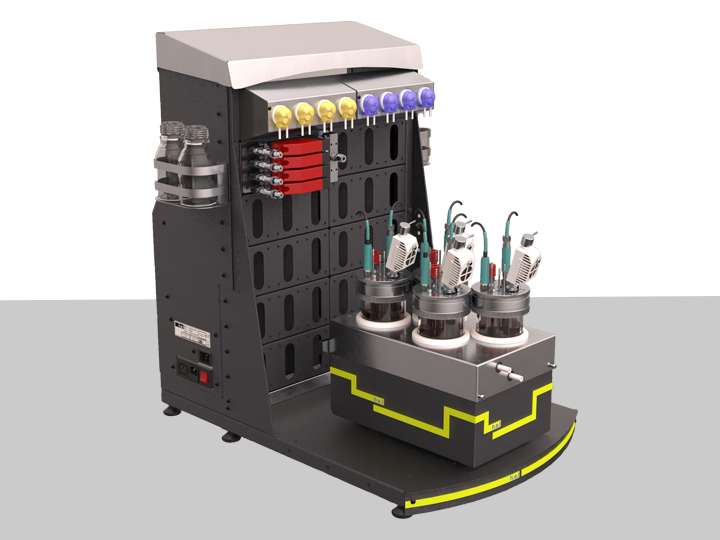
BioXplorer 400 | bench-top, parallel 4 bioreactor platform
The BioXplorer 400 is equipped with 4 bioreactors with working volumes of up to 400 ml. It...
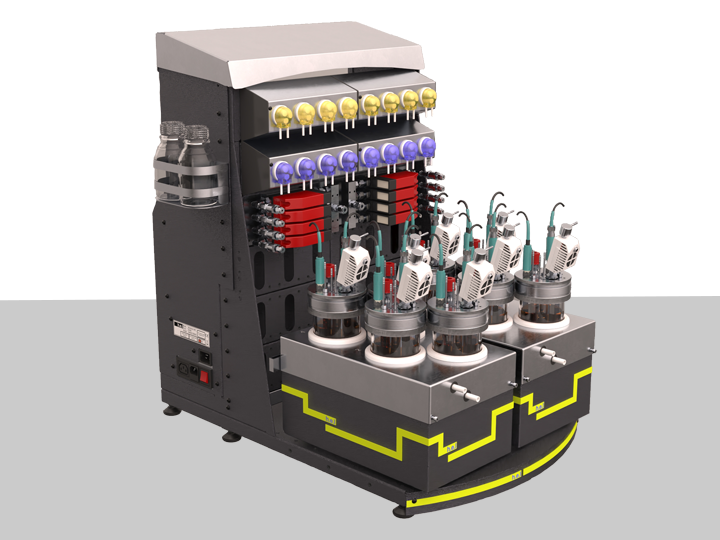
BioXplorer 400XL | Expanded Bench-Top, Parallel 8 Bioreactor Platform
Featuring eight 500 ml bioreactors, the BioXplorer 400XL allows the fast optimization of c...
How can the different processes be compared using bioreactors?
To improve bioprocesses, parallel experiments with different conditions, variants, and strategies are fundamental to finding the optimal conditions for cell growth, productivity, and/or product quality. This step is improved by the capacity of running several bioreactors at the same time, in which the different variables are modified to try to identify the right array of values. Additionally, bioreactors allow for the automatization of the process, increasing the statistical relevance of the results and decreasing the risks of human error.
Solutions
The BioXplorer is a powerful tool for screening processes. Its capabilities to control the different vessels (4 or 8, depending on the BioXplorer model) individually allow for evaluating different parameters, producing exceptionally accurate and detailed data. On the other hand, its integration with WinISO allows for the automatization of the process, reducing the risk of human error and the tight control of the conditions. This results in the generation of statistically robust data. Combining these capabilities, positions the BioXplorer as an invaluable tool for effective and efficient bioprocess screening.
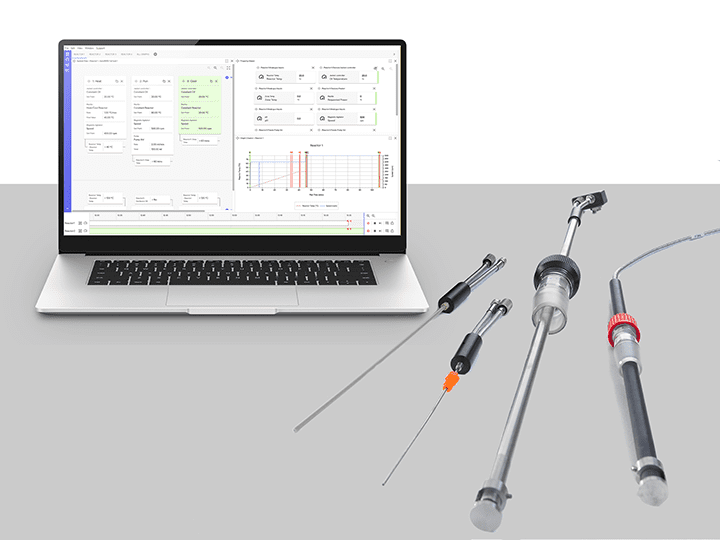
BioVIS | An Inline Cell Density Indicator Sensor
BioVIS is a probe for the inline monitoring of total cell growth and biomass within a bior...
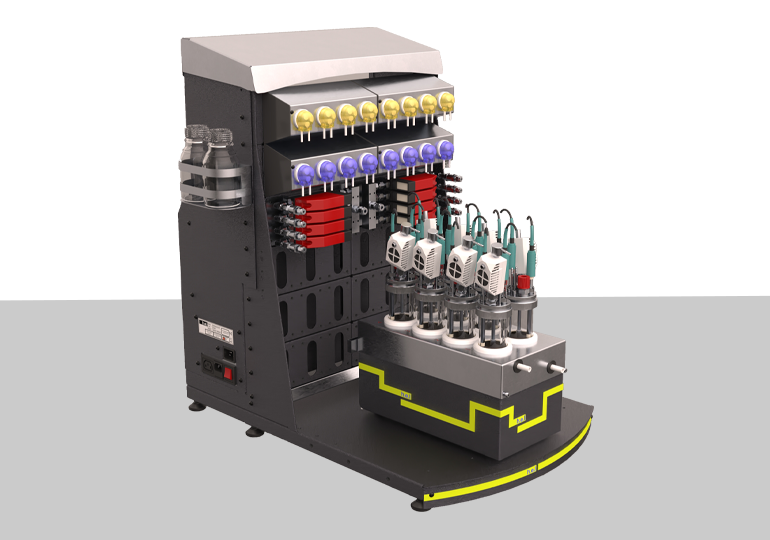
BioXplorer 100 | bench-top, parallel 8 bioreactor platform
The BioXplorer 100 is a multi-bioreactor system designed for the optimization of aerobic f...
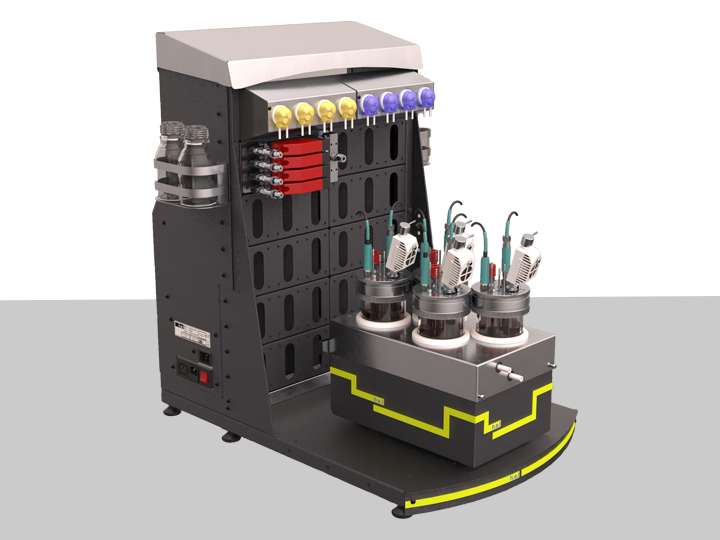
BioXplorer 400 | bench-top, parallel 4 bioreactor platform
The BioXplorer 400 is equipped with 4 bioreactors with working volumes of up to 400 ml. It...
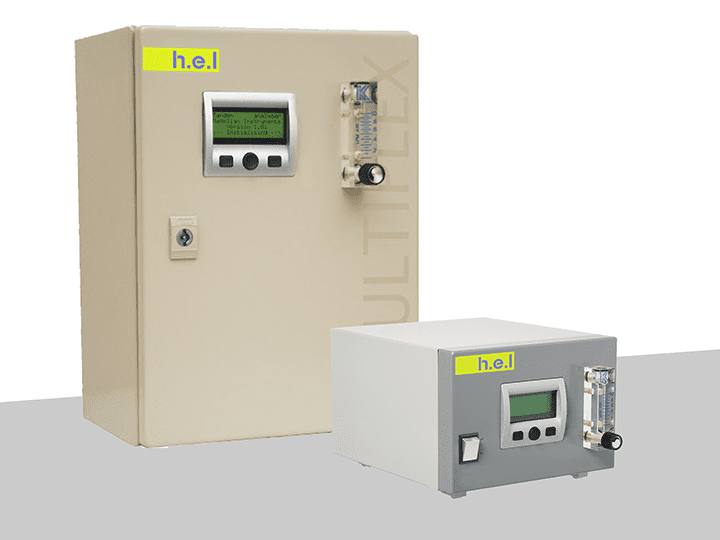
Tandem Off Gas Analyzers | Multi Line and Continuous Systems
The Tandem gas analyzer systems will give you real-time, on-line data that helps you under...
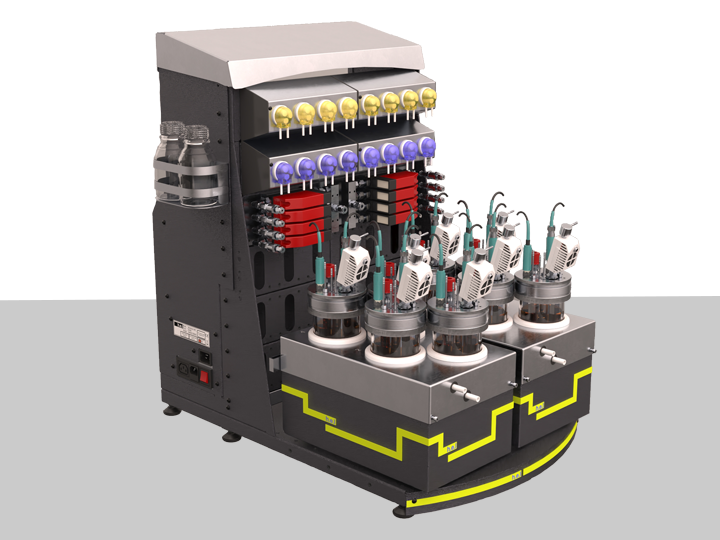
BioXplorer 400XL | Expanded Bench-Top, Parallel 8 Bioreactor Platform
Featuring eight 500 ml bioreactors, the BioXplorer 400XL allows the fast optimization of c...
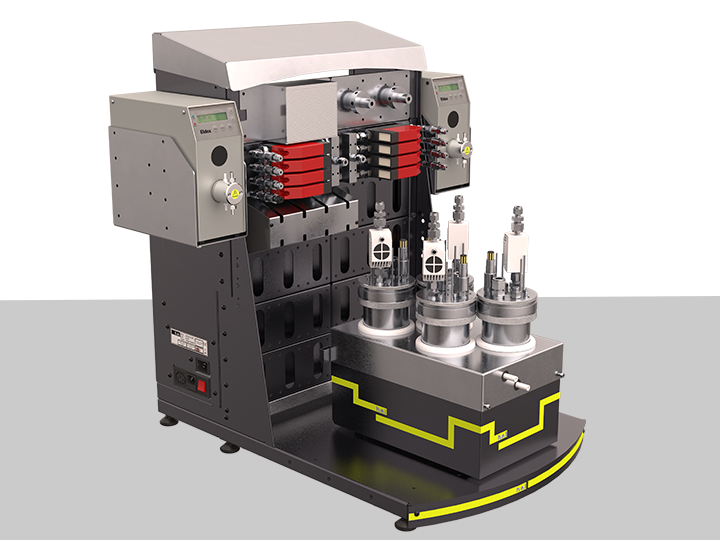
BioXplorer 400P | High-pressure bench-top, parallel 4 bioreactor platform
The BioXplorer 400P is an automated parallel pressure bioreactor system for the discovery ...
Process Development
What are the possible configurations that bioreactors can have?
Stirred-tank designs are probably the most common form of bioreactor, and they share several consistent design features with specific modifications that support a particular bioprocess. The main body of a reactor is a cylindrical vessel that contains the cells, culture media, and other components added to the reaction mix.
The most common materials for the bioreactor body are borosilicate glass or stainless steel. Borosilicate glass has the advantage of being highly durable and chemical resistant. Similarly, stainless steel bioreactors are durable and have low reactivity to chemicals and components typically used in bioprocesses. One advantage of stainless steel (generally 316L grade) designs is a greater tolerance to withstanding pressure differences. This pressure tolerance becomes especially important in large-scale vessels, where pressurized steam is used for Sterilization in Place (SIP) between individual bioprocess runs.
Some form of stirring device is required to mix all the culture media components, prevent cells from clumping together or settling to the bottom of the bioreactor, and promote mass transfer.
The agitator’s design should support the system’s overall design characteristics and the intended bioprocess. The agitator’s impeller portion can successfully use several designs, but it will either provide radial flow mixing or axial flow mixing. Radial flow impellers tend to have flat blades, and they will cause liquid flow around the radius of the bioreactor – a circular motion around the sides. Radial flow impellers are most appropriate when gas-liquid mixing or other blending is crucial. Axial flow impellers have pitched blades and will provide liquid flow along the bioreactor axis – flow between the tank’s top and bottom. Axial flow impellers give a gentler but more efficient mixing effect and are suited to bioprocesses with shear-sensitive cells or particles.
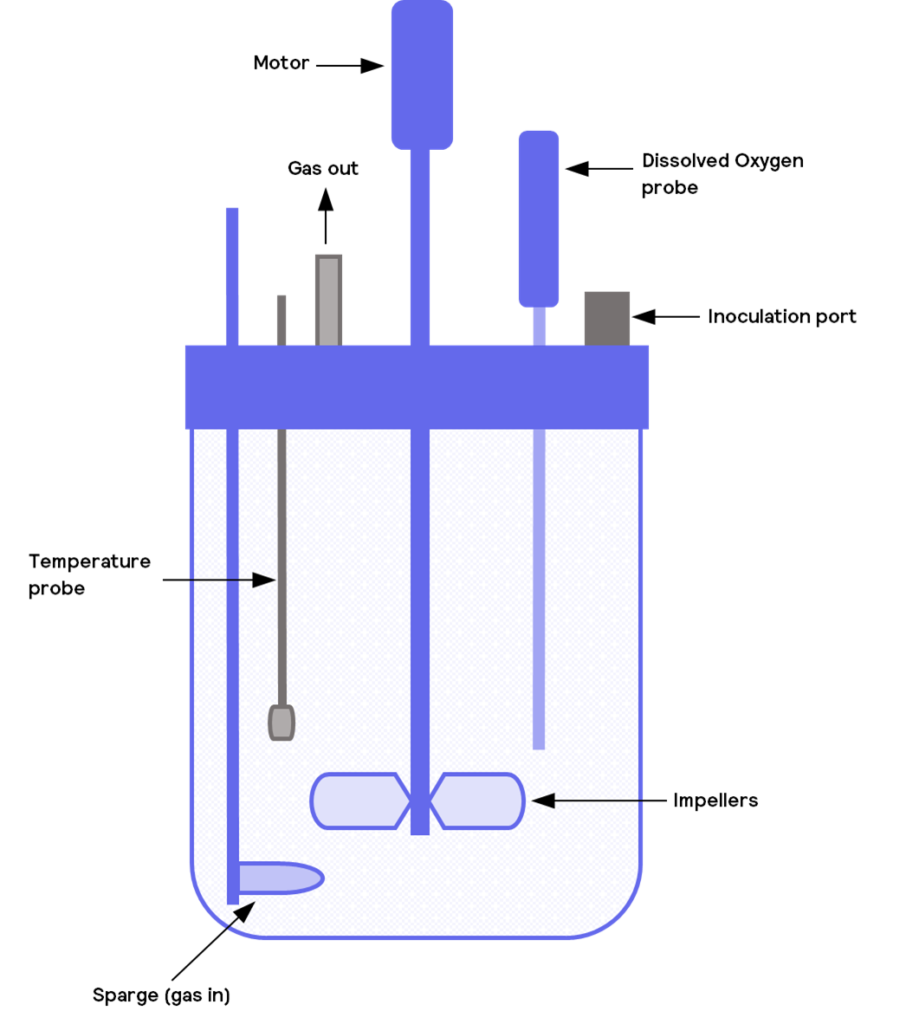
The agitator can be powered through a direct or an indirect linkage to a motor. In both cases, the motor is outside the bioreactor’s body. With a direct linkage, the motor is coupled directly to a stirrer shaft, which enters through the reactor vessel’s top or bottom. The impellor is then fixed onto that shaft. The motor is connected to a magnetic driver outside the bioreactor in an indirect linkage. The impeller sits entirely within the reactor vessel and has corresponding magnets that couple to the external driver. With indirect power, the impeller can be fitted to a stirrer shaft (the shaft contains the magnetic coupling), or the impeller can directly include magnets and be shaftless.
A direct coupling has the advantage that it can provide higher stirrer torque, so it is more suitable for viscous mixtures or when cells form mats or clumps. Indirect coupling tends to offer lower torque, and care must be taken to prevent the magnets from decoupling, such as running the stirrer too fast.
Inlets allow the introduction of fluid, such as fresh media, but can also include other sources, such as acid and bases. One of the critical parameters in biological processes is pH. The addition of pH probes in the bioreactor ensures close monitoring of its values and correction when a diversion from acceptable value happens. To correct this value, acids or bases can be added. Although these can all be provided manually, automated systems are commonly used based on either process knowledge or bioprocess monitoring. Automated systems connect inputs from the probes and measurements inside the reactor with specified bioprocess operating set-points with the pumps that will then feed in an appropriate liquid to maintain the optimum conditions.
Solutions
The BioXplorers and their versatility can play essential roles in Process Development. BioXplorers support a range of operational modes, including batch, fed-batch, and continuous reactors. They are highly versatile and allow for a range of measurements as standard, including temperature, pH, dissolved oxygen (DO), foam formation, liquid level, oxidation-reduction potential (ORP), and cell density. To prevent volume loss linked to evaporation, the BioXplorer is equipped with Peltier coolers, avoiding vapor loss through the gas line. This flexibility, combined with its versatile reactor configurations, makes it a dynamic solution in the advancement of bioprocess development. Furthermore, combined with WinISO, it permits the automatization of processes, increasing reliability and diminishing the chances of human error.
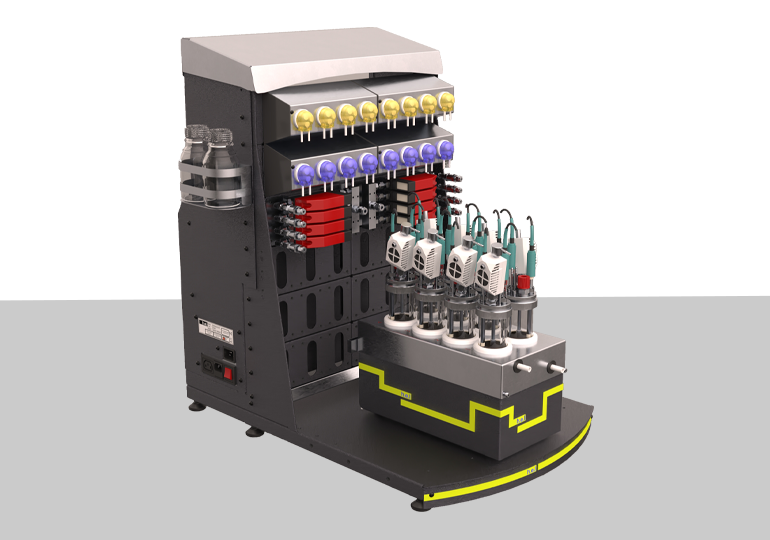
BioXplorer 100 | bench-top, parallel 8 bioreactor platform
The BioXplorer 100 is a multi-bioreactor system designed for the optimization of aerobic f...
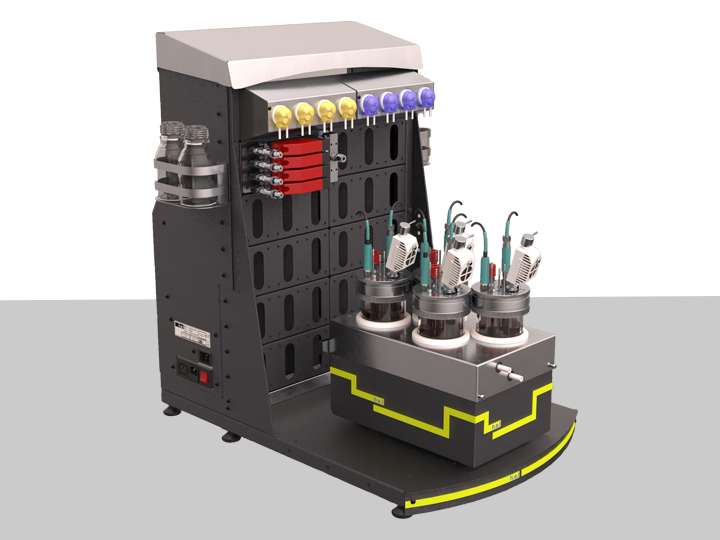
BioXplorer 400 | bench-top, parallel 4 bioreactor platform
The BioXplorer 400 is equipped with 4 bioreactors with working volumes of up to 400 ml. It...
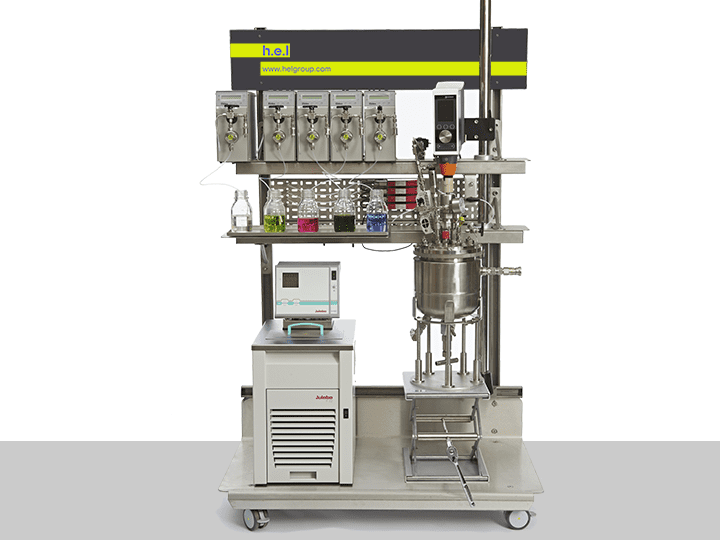
BioXplorer 5000 | Lab-scale bioreactor platform
The BioXplorer 5000 and 5000P single bioreactor systems are ideally suited to drive your s...
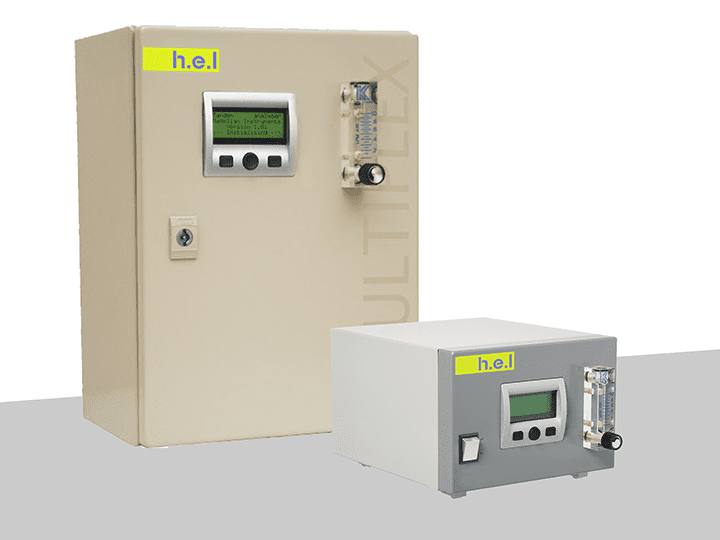
Tandem Off Gas Analyzers | Multi Line and Continuous Systems
The Tandem gas analyzer systems will give you real-time, on-line data that helps you under...
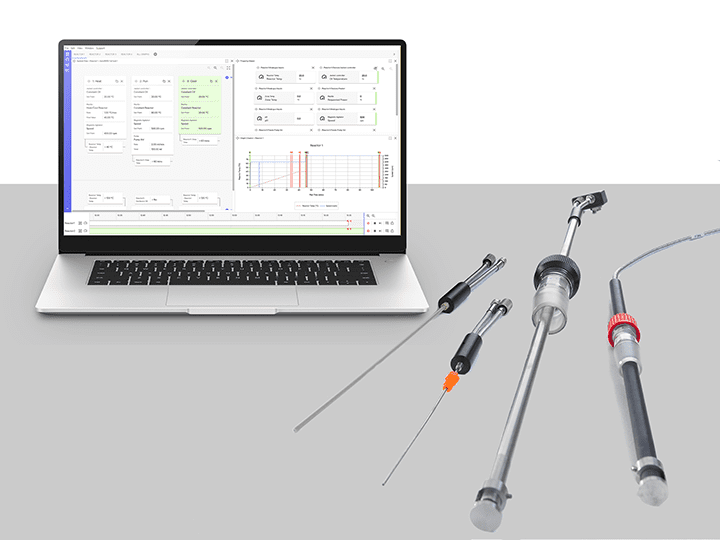
BioVIS | An Inline Cell Density Indicator Sensor
BioVIS is a probe for the inline monitoring of total cell growth and biomass within a bior...
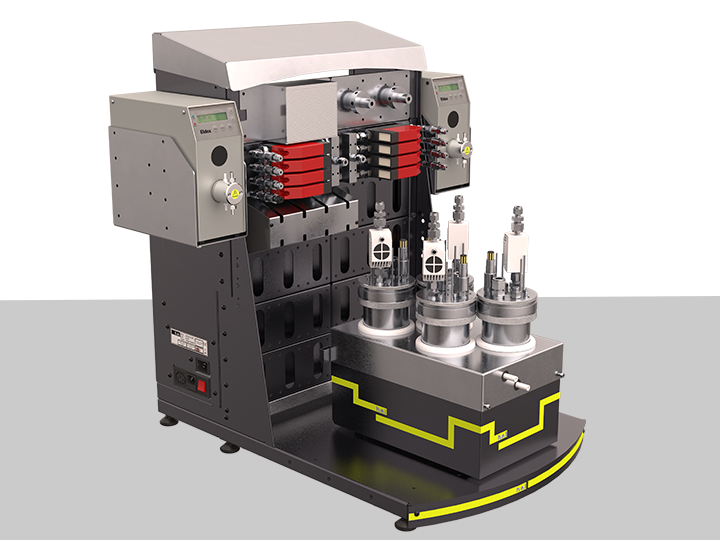
BioXplorer 400P | High-pressure bench-top, parallel 4 bioreactor platform
The BioXplorer 400P is an automated parallel pressure bioreactor system for the discovery ...
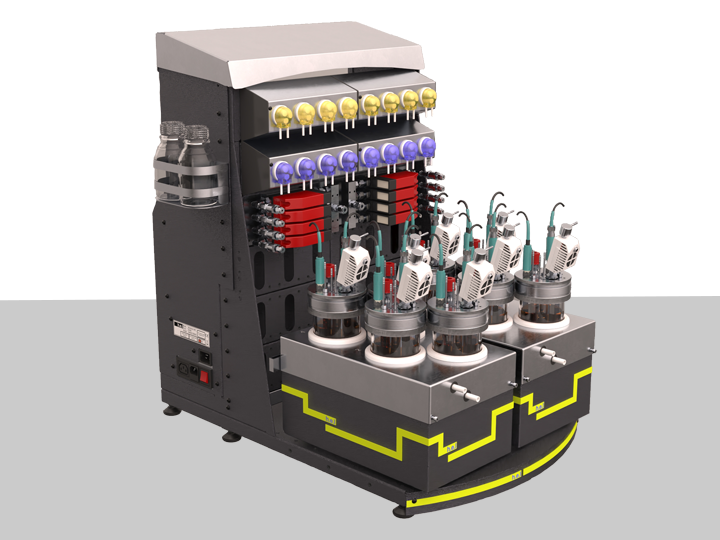
BioXplorer 400XL | Expanded Bench-Top, Parallel 8 Bioreactor Platform
Featuring eight 500 ml bioreactors, the BioXplorer 400XL allows the fast optimization of c...
Scale-Up
What needs verification before going into industrial scale?
Parameters such as temperature, pH, agitation, and aeration are fundamental and should have been optimized at a smaller scale. Pilot scale processes are an invaluable tool and act as a bridge between laboratory scale and plant scale. However, larger volumes can decrease the efficiency of mass and energy transfer, potentially impacting the productivity and effectiveness of the overall process. This allows for verification that the previous optimization is still valid and, if not, gives the opportunity to tune the variables that result in underperformance. One of the critical aspects to evaluate at the pilot scale is aeration and mixing, which can become challenging at increased volumes, resulting in non-uniform distribution of nutrients and oxygen. This can result in decreased cell growth and product formation.
Pilot scale studies can help to create conditions that mimic the production scale process through testing and refinement. Achieving these conditions can facilitate a smoother transition to industrial-scale production. Moreover, rigorous testing can minimize risk operational and financial setbacks due to unanticipated problems once in full production.
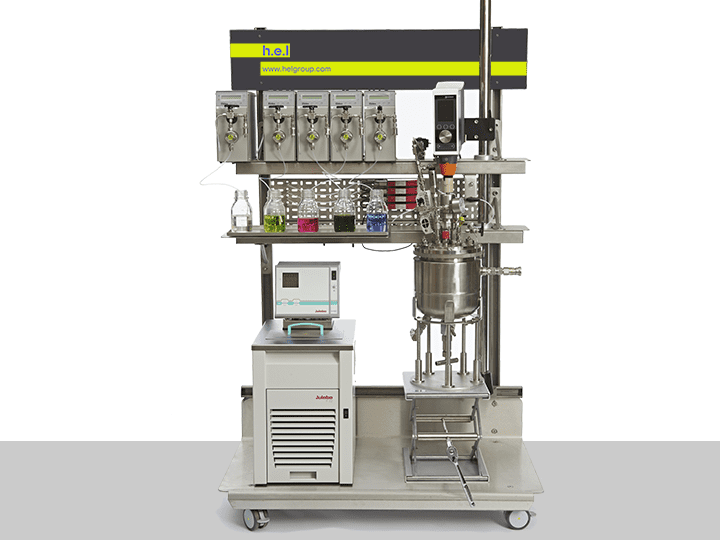
BioXplorer 5000 | Lab-scale bioreactor platform
The BioXplorer 5000 and 5000P single bioreactor systems are ideally suited to drive your s...
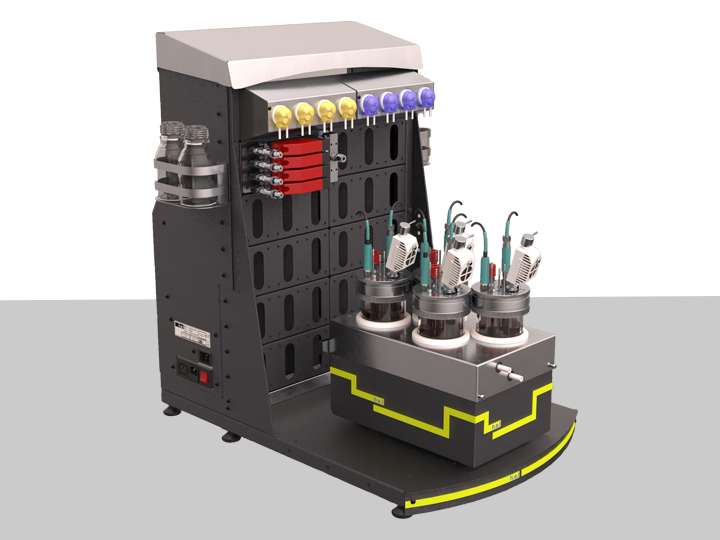
BioXplorer 400 | bench-top, parallel 4 bioreactor platform
The BioXplorer 400 is equipped with 4 bioreactors with working volumes of up to 400 ml. It...
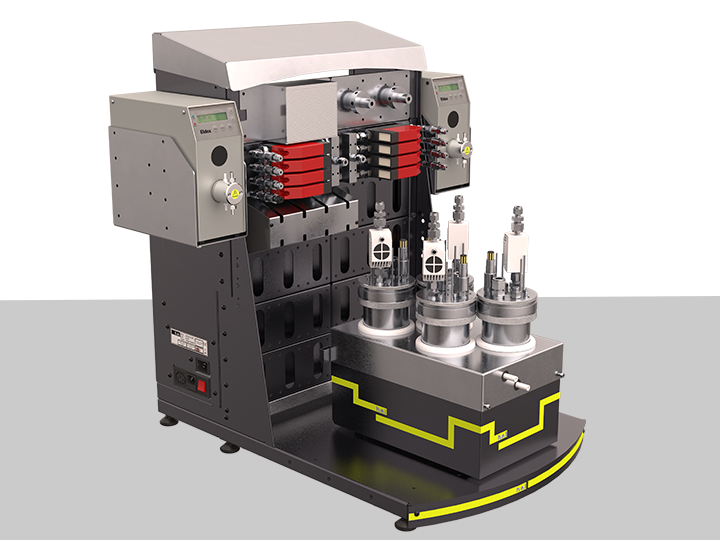
BioXplorer 400P | High-pressure bench-top, parallel 4 bioreactor platform
The BioXplorer 400P is an automated parallel pressure bioreactor system for the discovery ...
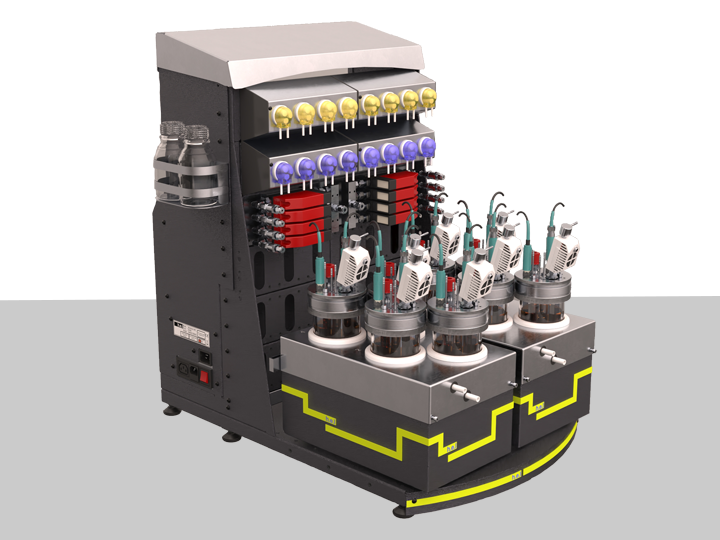
BioXplorer 400XL | Expanded Bench-Top, Parallel 8 Bioreactor Platform
Featuring eight 500 ml bioreactors, the BioXplorer 400XL allows the fast optimization of c...
How do we ensure the consistent quality of the product?
Benchtop and pilot scale experiments can be used to demonstrate the reproducibility of the biological process. Additionally, due to their smaller size, several bioreactors can be run in parallel to check whether the data produced is consistent. This stage is fundamental in verifying the robustness of the process, which is a crucial requirement when adhering to Good Manufacturing Practices (GMP).
At this stage, numerous batches must be run to demonstrate reproducible and consistent results in yield, product quality, and other critical characteristics under defined operating conditions. Reproducible processes at the pilot scale increase confidence for the industrial scale and are particularly relevant for regulatory submission.
Consistency, on the other hand, refers to the ability of the bioprocess to generate a product of uniform quality. On a pilot scale, the quality of the product is monitored throughout batches to ensure that parameters such as purity and potency remain constant. This is challenging to the inherent variability of biological systems, but by using strict control and parameters and consistent substrates, this variability can be kept to a minimum.
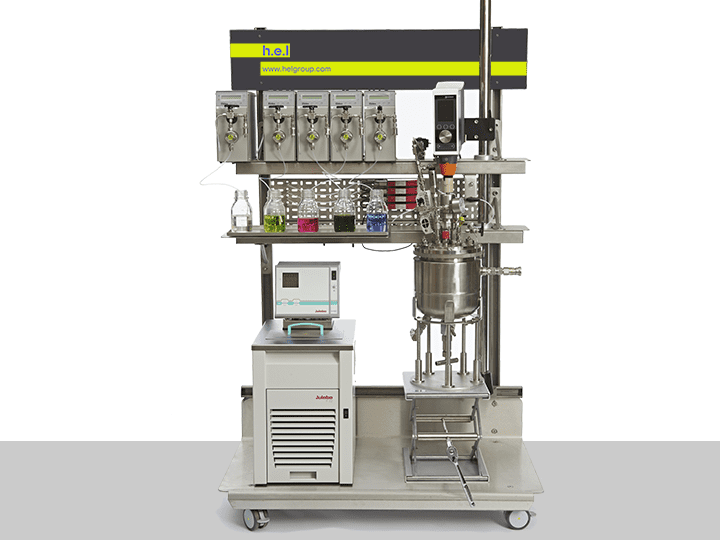
BioXplorer 5000 | Lab-scale bioreactor platform
The BioXplorer 5000 and 5000P single bioreactor systems are ideally suited to drive your s...
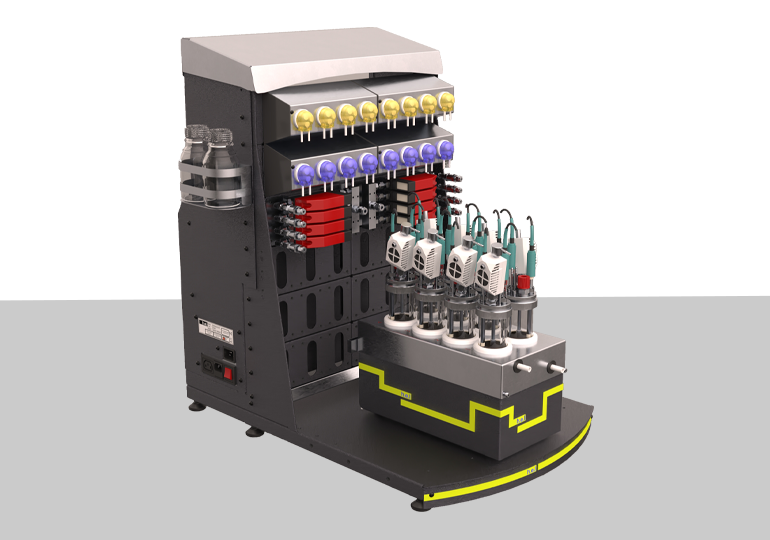
BioXplorer 100 | bench-top, parallel 8 bioreactor platform
The BioXplorer 100 is a multi-bioreactor system designed for the optimization of aerobic f...
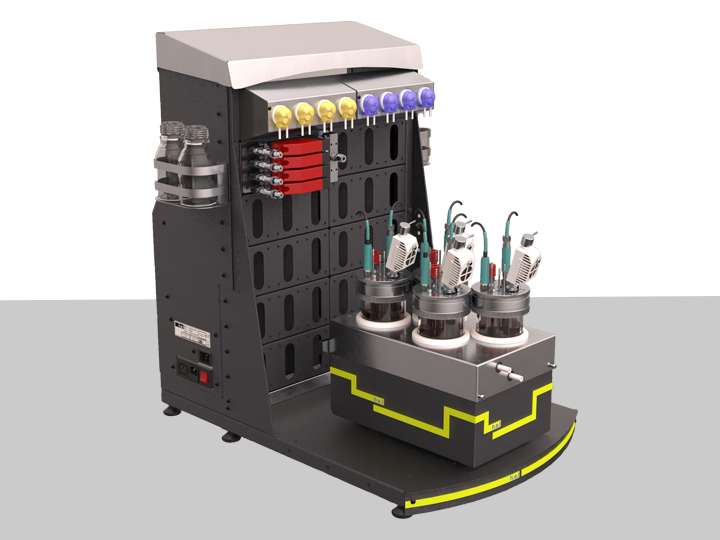
BioXplorer 400 | bench-top, parallel 4 bioreactor platform
The BioXplorer 400 is equipped with 4 bioreactors with working volumes of up to 400 ml. It...
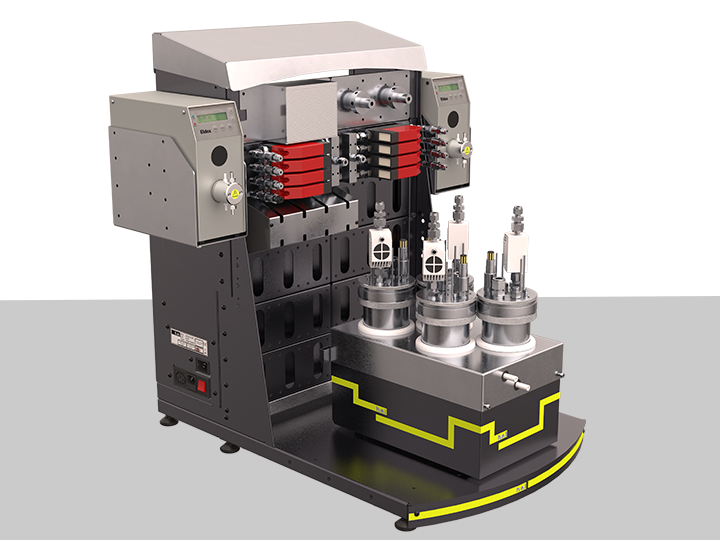
BioXplorer 400P | High-pressure bench-top, parallel 4 bioreactor platform
The BioXplorer 400P is an automated parallel pressure bioreactor system for the discovery ...
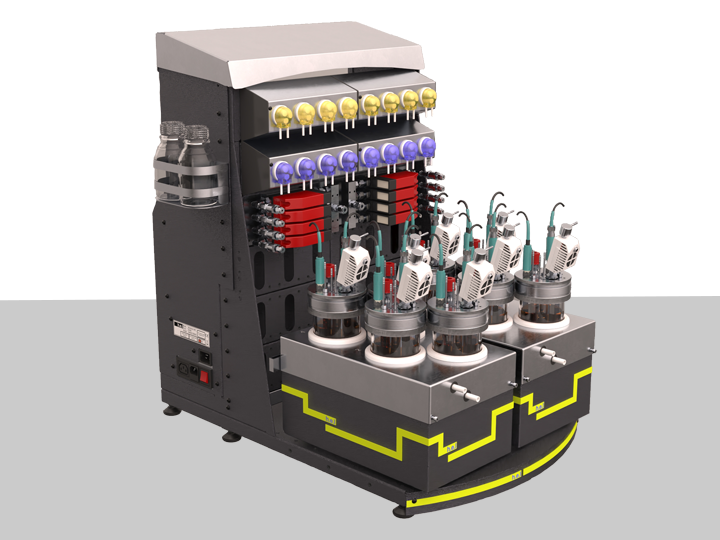
BioXplorer 400XL | Expanded Bench-Top, Parallel 8 Bioreactor Platform
Featuring eight 500 ml bioreactors, the BioXplorer 400XL allows the fast optimization of c...
How can the pilot scale help reduce future risks?
At a pilot scale state, bioprocesses are tested under conditions that mimic industrial-scale operation. However, their smaller size offers a more manageable and controllable environment. This can provide a safety net; if issues arise, they can be addressed more cost-efficiently than at a full industrial scale. Thus, the pilot scale can serve as a crucial checkpoint, allowing for the early identification and correction of problems.
The pilot scale also can function as the training ground for operators, allowing them to familiarize themselves with the intricacies of process dynamics, equipment, and controls. This familiarization process can be helpful and reduce the risk of operation error, leading to safety incidents at full-scale production. Additionally, the data generated during the pilot stage can be used to validate the process.
Solutions
The BioXplorer, especially the BioXplorer 5000, is an excellent platform for scale-up processes. This model can operate at regular or high pressure and up to 5 L volumes. This facilitates a comprehensive understanding of critical processes that can be affected during scaling-up, such as energy and mass transfer at a larger scale. Moreover, it enables the exploration of oxygen and nutrient distribution dynamics, proving its worth as a versatile tool for optimization.
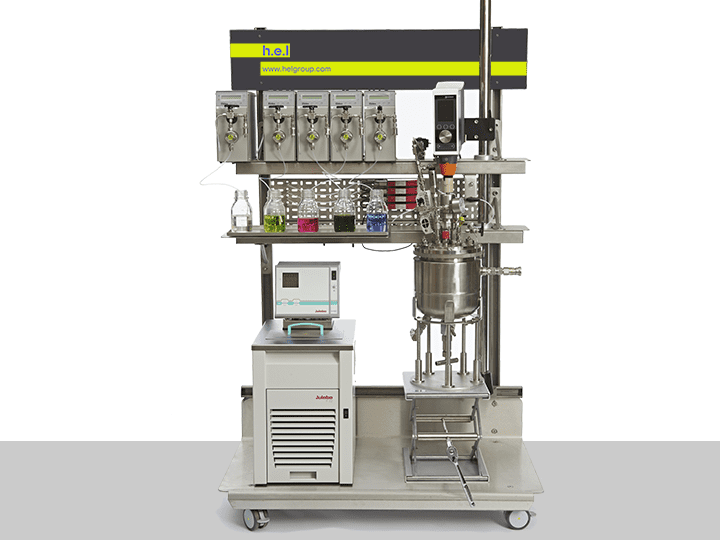
BioXplorer 5000 | Lab-scale bioreactor platform
The BioXplorer 5000 and 5000P single bioreactor systems are ideally suited to drive your s...
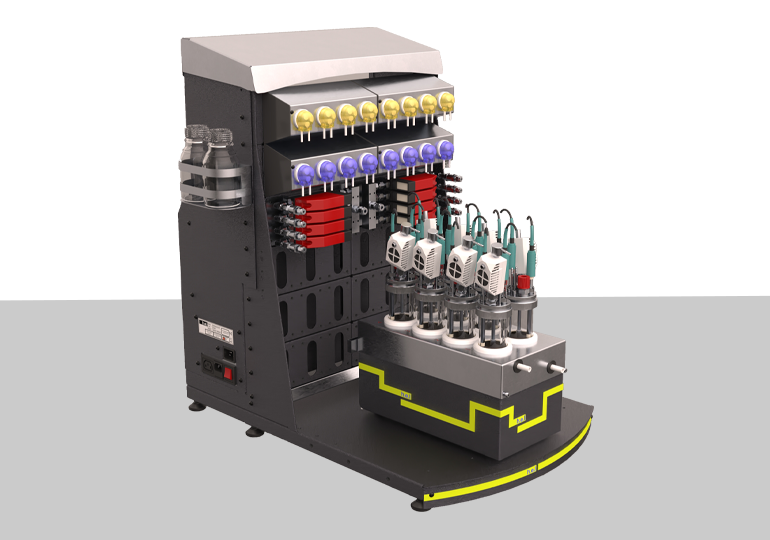
BioXplorer 100 | bench-top, parallel 8 bioreactor platform
The BioXplorer 100 is a multi-bioreactor system designed for the optimization of aerobic f...
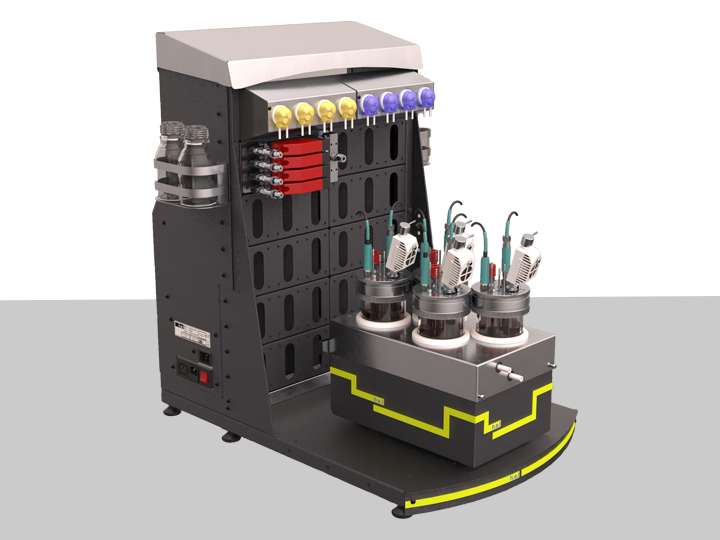
BioXplorer 400 | bench-top, parallel 4 bioreactor platform
The BioXplorer 400 is equipped with 4 bioreactors with working volumes of up to 400 ml. It...
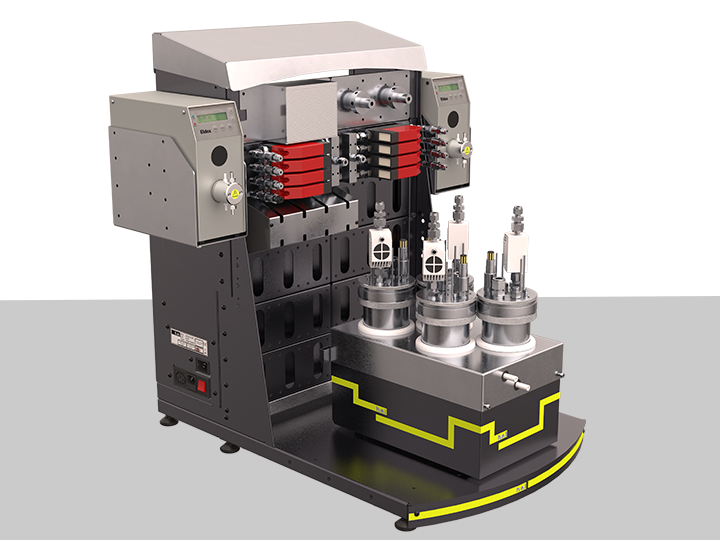
BioXplorer 400P | High-pressure bench-top, parallel 4 bioreactor platform
The BioXplorer 400P is an automated parallel pressure bioreactor system for the discovery ...
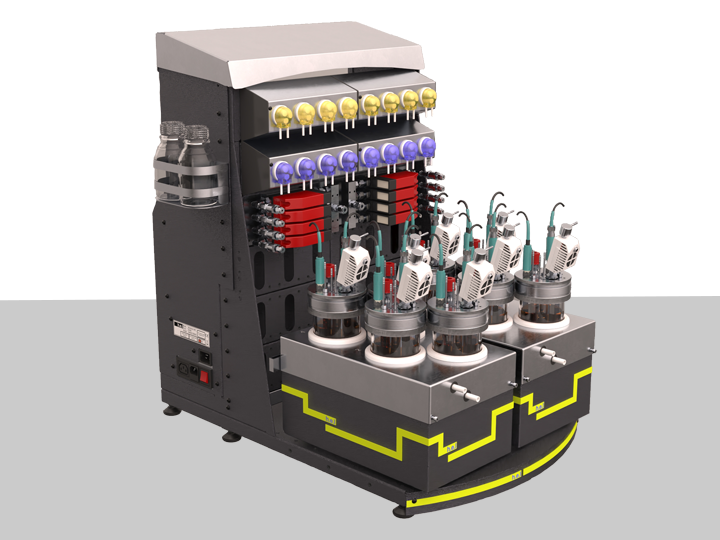
BioXplorer 400XL | Expanded Bench-Top, Parallel 8 Bioreactor Platform
Featuring eight 500 ml bioreactors, the BioXplorer 400XL allows the fast optimization of c...
Scale Down
Why are scaling down processes important?
Scale-down process plays a crucial role in the bioprocess industry for a number of reasons:
Process Development and Optimization: scale down processes because their capabilities to recreate large processes at a smaller scale result in cost-effective and rapid ways to test conditions, such as growth media, temperature, pH, or oxygen scale.
Understanding Process Challenges: large-scale reactors face typical problems, such as mixing or aeration, which can be overseen in the process development. These issues can significantly impact industrial production, but scale-down processes can help identify these potential challenges.
Regulatory requirements: regulatory authorities require a demonstration of process understanding and control in manufacturing. Scale-down models contribute to proving this understanding as part of the quality by design.
Two approaches arise in recreating the physical, chemical, and biological parameters that cells encounter at a large scale in small reactors.
Statistical scale-down
This approach aims to identify the critical factors influencing the process outcome and simulate their effects using statistical analysis. In this type of scale-down, lab-scale reactors are used to perform a large number of experiments to generate a matrix of variables corresponding to conditions that affect the performance of the bioprocess. This method is less time-consuming and costly, but on the other hand, it can lack the fidelity of a physical scale-down.
Generally, statistical scale-down involves designing experiments that will capture the variability of large-scale processes, followed by data collection of both the variable conditions and response variables. Based on the data, a statistical model can be constructed describing the process performance, which will finally be validated based on the accuracy of its predictions.
Physical scale-down
This approach aims to simulate large-scale conditions, mimicking heterogeneous conditions encountered in small-scale reactors. To achieve this, laboratory-scale bioreactors are engineered. These smaller-scale reactors reproduce environmental conditions and simulated physicochemical parameters, such as gradients, which will introduce heterogeneity using approaches like intermittent feed or pulsing air flow. Detailed monitoring of cell behaviors will show how they react to the different conditions in the reactor and how they affect growth, product formation, and nutrient consumption, among other parameters. The results obtained from physical scale-down will then go through an iterative optimization process, helping to improve the efficiency of the process.
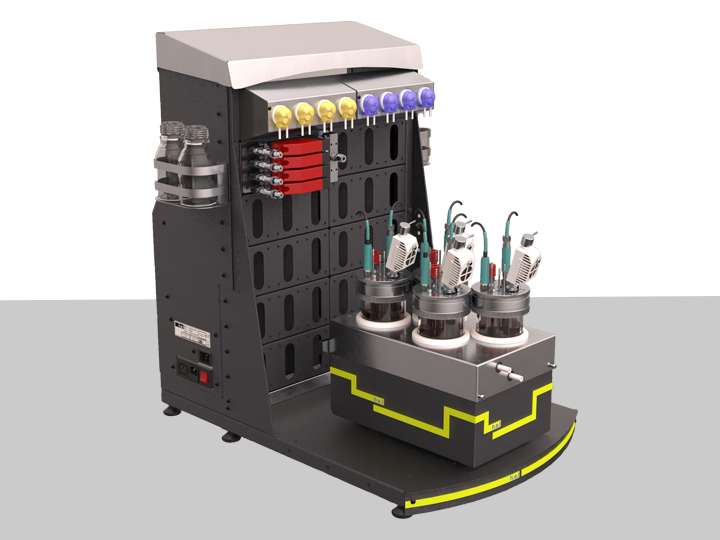
BioXplorer 400 | bench-top, parallel 4 bioreactor platform
The BioXplorer 400 is equipped with 4 bioreactors with working volumes of up to 400 ml. It...
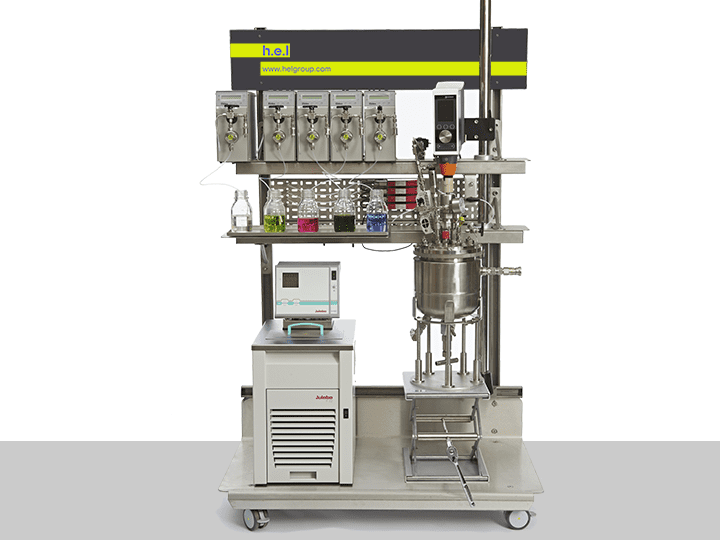
BioXplorer 5000 | Lab-scale bioreactor platform
The BioXplorer 5000 and 5000P single bioreactor systems are ideally suited to drive your s...
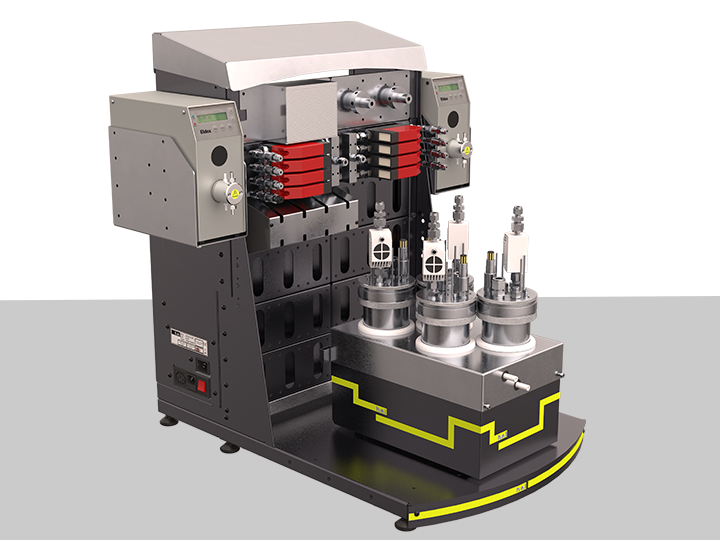
BioXplorer 400P | High-pressure bench-top, parallel 4 bioreactor platform
The BioXplorer 400P is an automated parallel pressure bioreactor system for the discovery ...
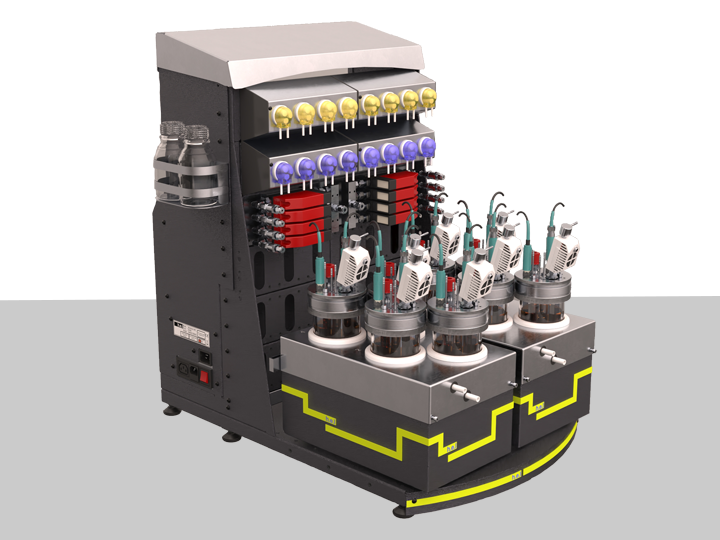
BioXplorer 400XL | Expanded Bench-Top, Parallel 8 Bioreactor Platform
Featuring eight 500 ml bioreactors, the BioXplorer 400XL allows the fast optimization of c...
What challenges does the process of scale-down face?
Recreating conditions as close to reality as possible in small reactors can be challenging due to the wide range of complex variables involved.
For instance, reproducing gradients that may occur in large-scale reactions due to the imperfect mix can be complex, as small-scale reactors are often well mixed, resulting in relatively homogeneous conditions. This links to another potential challenge faced by agitation at the time of down-scaling. Mixing and stirring in large-scale reactors often rely on the use of a large impeller, which will ensure adequate mixing, therefore ensuring appropriate mass and energy transfer rates. On the downside, these large impellers can exert shear stress on the cells, so balancing both values can prove difficult. Scale-down models, especially physical ones, can be time- and resource-consuming because of the time spent carefully controlling numerous parameters.
Additionally, the iterative nature also adds to the time and cost involved. However, one of the biggest challenges these tests can face is representing biological complexity, which is very difficult to capture into a scale-down model due to the highly variable nature of biological systems. Apart from biological complexity, the temporal complexity of the system can also be challenging to reproduce due to the often transient conditions changes.
Solutions
BioXplorers can play a fundamental role in scale-down process analysis. Using H.E.L’s WinISO, BioXplorer 100 and 400 can be finely controlled to replicate the conditions found at a larger scale accurately. Multiple reactors can be used to increase the throughput of the process, positioning them as a potent tool for statistical scale-down processes. On the other hand, larger reactors, such as BioXplorer 5000, can be used to replicate complex conditions, such as physicochemical gradients, and therefore can be instrumental in physical scale-down processes. The application of scale-down processes using BioXplorer models results in significant time and cost reductions, underscoring their importance in efficient and economical bioprocess management.
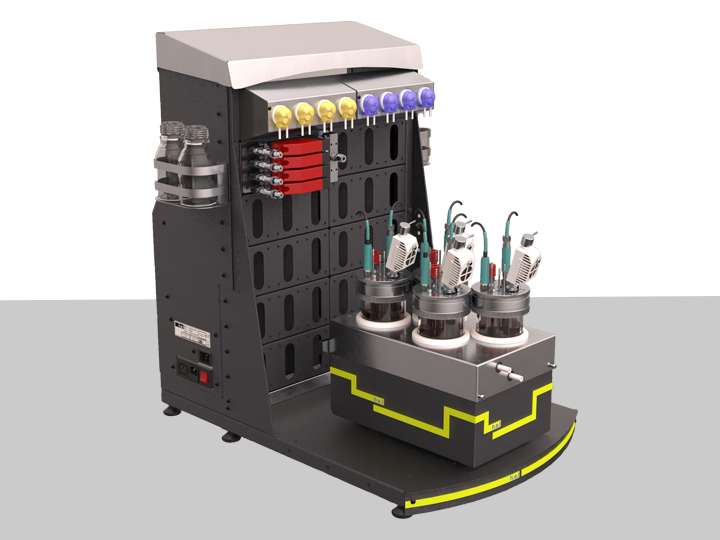
BioXplorer 400 | bench-top, parallel 4 bioreactor platform
The BioXplorer 400 is equipped with 4 bioreactors with working volumes of up to 400 ml. It...
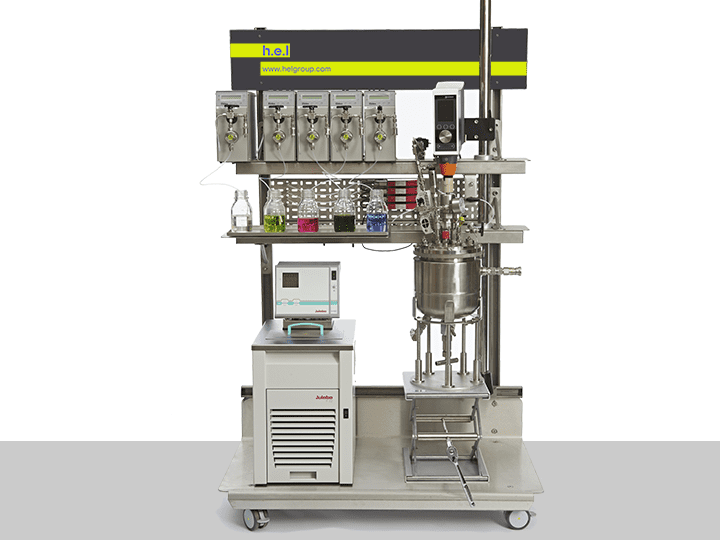
BioXplorer 5000 | Lab-scale bioreactor platform
The BioXplorer 5000 and 5000P single bioreactor systems are ideally suited to drive your s...
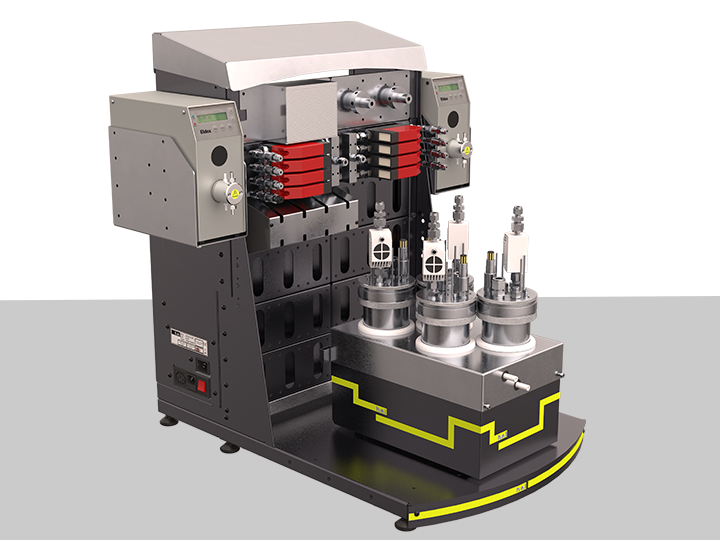
BioXplorer 400P | High-pressure bench-top, parallel 4 bioreactor platform
The BioXplorer 400P is an automated parallel pressure bioreactor system for the discovery ...
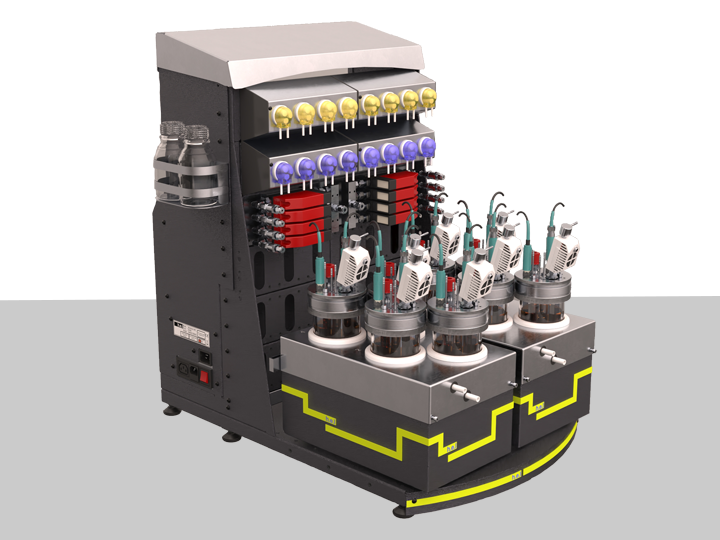
BioXplorer 400XL | Expanded Bench-Top, Parallel 8 Bioreactor Platform
Featuring eight 500 ml bioreactors, the BioXplorer 400XL allows the fast optimization of c...